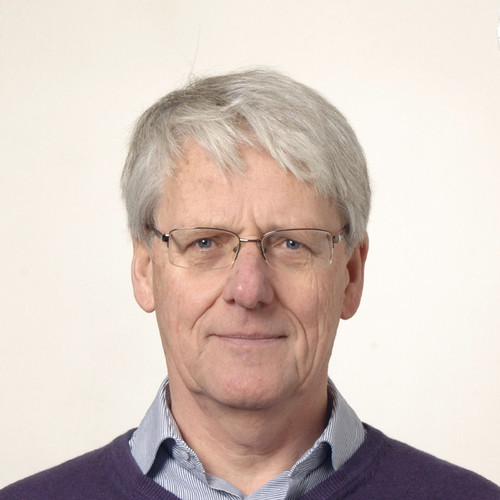
Teun Klapwijk spoke on energy-resolving THz detectors for astrophysical
observations. These frequencies are of interest for learning about the early
universe, some 400 million years ago, where radiation from the first stars
excites nearby dust, which then re-radiates in the THz band (~100-600 micron
wavelength).
Previous cryogenic detectors used for this purpose are TESs, photon-assisted
tunneling devices (SIS junctions), and HEB mixers.
in photon-assisted tunneling detectors, an SIS junction is voltage biased
such that THz photons can create quasiparticles above the gap by direct
absorption. HEB mixers use the self-heating-induced nonlinearity in a
nanoscopic superconducting microbridge to mix THz radiation down to
microwave frequencies. TESs use a very sharp resistive transition in a very
low-Tc material as a thermometer to detect the temperature change of a very
low heat-capacity, well-isolated absorber due to the absorption of single
photons.
TESs are the most advanced of these and have the world-record low
noise-equivalent power of ~10^-20 W/Sqrt[Hz] (Gershenson). This has now
reached the limit associated with the background photons, below which
further increases are less useful.
However, one disadvantage of these detectors is that they are read out with
SQUID amplifiers, which are difficult to read out in extremely large
numbers. The most advanced TES array for this purpose, SCUBA II, has 10000
array elements.
Microwave kinetic-inductance detectors have a possibility to go beyond this
limitation. They are built out of extremely high-Q resonators of disordered
superconducting films, with high kinetic inductance and broadband
absorption. Absorption of a photon generates quasiparticles which both shift
the resonator frequency (due to the modified kinetic inductance) and reduce
the Q (due to additional dissipation). This can be detected my monitoring
the microwaves reflected from the resonator. In addition, many resonators
can be connected to the same feedline in parallel, each with a slightly
different resonance frequency. As the Q is increased, the density of these
frequencies can also be increased, up to the required bandwidth for the
detector elements. This allows many detector elements to be read out on a
single coax, so that very large arrays can be contemplated without requiring
impractically many coaxes.
The figure of merit for an MKID involves several factors. First, the
fraction of the total inductance in the resonator which is kinetic; ideally
this would be 1 for maximum sensitivity, and this suggests the use of very
high kinetic inductance materials and geometries. Second, the quasiparticle
recombination time, which should be as long as possible, such that a given
number of excitations can be measured for maximum time in the resonator.
Third, the resolator Q should be as high as possible, in order to be
sensitive to as small an inductance change as possible. Finally, the
quasiparticle density of states should be as low as possible, for maximum
fraction change in the kinetic inductance for a given number of
quasiparticles.
TiN maximizes these figures of merit in many ways better than any material
previously tried:
- The high sheet resistance corresponds to a very high kinetic inductance,
giving kinetic inductance fractions near unity, and very compact resonators.
- Extremely high Q values up to 2 x 10^7 have been demonstrated at high
power
- The Tc can be continuously tuned by varying the deposition parameters;
this allows low gaps to be used for maximum sensitivity
- The extreme disorder gives very good far-IR absorption
- In spite of the high disorder, the quasiparticle lifetime is still
reasonably long
Blogged by Jamie Kerman
No comments:
Post a Comment